Terahertz Technology Is Set To Enable Higher Performance In A Wide Range Of Applications
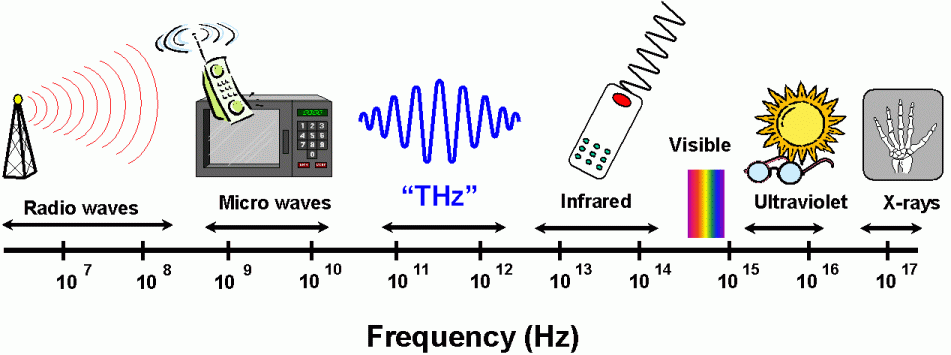
Developing sources and detectors of electromagnetic radiation has been fundamental to scientific progress. However, such devices are lacking in one a part of the spectrum – thus referred to as 'terahertz gap'. This frequency range, where no practical technologies for generating and detecting the THz radiation exist, lies between the microwave and infrared, covers frequencies from 100GHz to 10THz.
The THz gap matters because radiation from this part of the spectrum has unique features. So called T-rays have remarkable properties: they are intrinsically safe, non ionizing, non destructive. Detecting reflected THz radiation makes it possible to create spectroscopic information and 3D images with unique spectroscopic signatures – terahertz fingerprints – not found at alternative wavelengths like optical and infrared. THz imaging also produces results much more quickly than X-rays.
That is why its potential applications range so widely – from medical imaging, biological research, pharmaceutical monitoring and semiconductor testing to security, communications, manufacturing and quality control.
Science has used THz detection for decades to research things like dust in our galaxy and in telescopes like those at Mauna Kea and the Herschel Space Observatory. However, these are multi million dollar projects, using extremely specialized, customized THz systems, often involving unprecedented cooling – way far from the commercial world.
The conventional electronic sources and detectors used for radio and microwaves, which are powerful, compact, easy to use at room temperature and affordable, remain stubbornly difficult to produce for the THz band.
But things are improving and the field of THz photonics is growing in importance. This explains the emergence of several companies in the field.
An academic who agrees is Daniel Mittleman, Professor in the Electrical and Computer Engineering department at Rice University in the US. He names several application areas where THz technologies now has huge potential. First is the enhancement of techniques for table top generation of very high intensity THz pulses.
"Peak fields of 1MV/cm are not unusual any more. This opens up an entirely new realm of THz nonlinear optics, with huge impact in condensed matter spectroscopy, for example."
Secondly, there is the future of wireless communications.
"It is inevitable that consumer accessible wireless networks will operate at more than 100GHz," he says. "Although 60GHz is already in production, the technologies required for frequencies in the range from 100 to 400GHz are going to look very different."
A main reason for the growth in THz photonics is semiconductor technology, notably the improving capabilities of silicon CMOS devices at higher frequencies.
"If you can replace a $100,000 laser with a $1 silicon chip that does the same thing, then the world will beat a path to your door. The technology is not quite there yet. But I envision Moore's law will continue to carry CMOS technology to higher frequencies and higher power, and it will have a growing impact on the THz world in the years to come."
And finally, THz imaging cameras are coming of age – megapixel focal plane arrays with fast processing capabilities.
"For many years, this was a 'Holy Grail', and now it's here," Mittleman says. "They are still too expensive, of course, but that will change in time. As the price falls, this is going to have a huge impact on many of the proposed applications, especially in security and sensing."
A major source of THz radiation is the quantum cascade laser (QCL), which typically operates between 2 and 5THz and is revolutionising the THz field. Previous THz sources were broadband, time domain based systems using a femtosecond, mode locked laser, which excited a photoconductive switch to generate the THz radiation. But these have drawbacks, like high cost and their relative low power. The QCL was the first semiconductor device that could emit high power, narrowband THz radiation.
The THz QCL appeared from research in Cambridge by Professors Giles Davies and Edmund Linfield, both now at Leeds University, which is a leading centre for THz R&D in the UK. Unlike typical semiconductor lasers, that emit through the recombination of electron–hole pairs across the material band gap, the laser emission by QCLs is achieved by exploiting phenomena that emerge from a repeated stack of semiconductor multiple quantum well heterostructures.
"The QCL is a really nice example of theoretical physics working," says Paul Dean, EPSRC Research Fellow at Leeds University. "Their operation involves complex quantum mechanics regarding things like the electron transport. So, in order to design them, you need sophisticated modelling tools.
The THz QCL hasn't reached full commercialisation yet because it still requires cryogenic cooling, restricting use in many industrial applications. If they could work at room temperature, it would make a big difference."
But progress is being done, due to improved designs and better control of the molecular beam epitaxy process for producing the semiconductors. This has enabled to develop THz systems capable of working at temperatures of more than 77K. The importance of this development is that liquid nitrogen can be used for cooling, rather than helium. This reduces the cost drastically – from more than £1 per litre to a fraction of a penny – and makes the whole process easier. Many standard tools, such as MRI scanners, use liquid nitrogen cooling.
Another example of room temperature operating generator comes from Terasense, produced with an IMPATT technology, compact and low cost. More info.
While generating THz radiation has been one technical challenge, detecting it has been another because THz photons carry about 100 times less energy than those of visible light, making them harder to detect. A lot of thermal processes happening in the detectors can cause problems, therefore the powerful cooling is needed.
One detector innovation developed recently is to use the QCL as both source and detector. The THz radiation from the laser is reflected by the external target and goes back into the laser.
"Just by measuring the voltage across the laser, you can measure quite accurately the radiation coming off your target. That has simplified things because instead of systems requiring the laser and a cryogenically cooled detector, you only need a laser with some simple focusing optics. We are now looking to explore the commercialisation of this," Dean says.
Another advance towards compact, sensitive and fast THz detectors was announced recently by Terasense researchers. Plasmonic detectors proposed by Terasense have good sensitivity comparable with other available detectors working in sub-THz range (0.05 – 1.0 THz) where most common materials are transparent. Detectors can be easily produced in large quantities in form of 2D array on 4” GaAs wafers, thanks to compatibility of the Terasense technology with mass semiconductor manufacturing lines, and assembled as THz imaging cameras.
The nanodetectors can handle large area, fast imaging across both THz and sub THz spectra, making possible a range of spectroscopic and real time imaging applications – and possibly fast megapixel THz cameras.
Technical advances have recently centred on improvements in detector sensitivities, helped by enhancements in semiconductor technology.